Overview | Energy Systems | Solar Fuel Generation | Light Directed Growth
Goal
Make arrays of three-dimensionally structured semiconductors that: 1) maximize absorption of light; and, 2) increase the probability of collecting the oppositely charged carriers generated by the absorption of light; while, 3) minimizing the total volume of the semiconducting material.
Strategy
In the bottom-up approach, we allow interactions between light and semiconductors to direct the growth of three-dimensional structures. Since in this case the growth is a response to the presence of light, the resulting structure is self-optimized for light absorption. We use photoelectrodeposition to grow the structures from chalcogen oxides dissolved in solution. We develop and use optical and materials models to understand how interactions between the light and the materials result in the three-dimensional structures we observe.
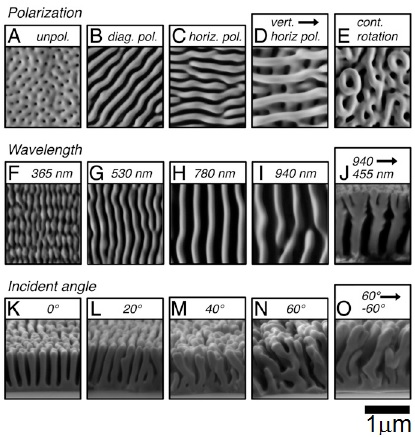
Scanning electron micrographs of selenium-tellurium films produced using light-directed growth showing effects of polarization, wavelength, and incident angle on the resulting structure.2
Highlights
Effects of Spectral Distribution and Polarization of Optical Inputs on Light-Directed Growth of Selenium-Tellurium Films
Selenium–tellurium films grown via electrodeposition from solutions containing a mixture of selenium oxide and tellurium oxide spontaneously develop ordered, lamellar patterns in response to the presence oflight.2 The space between the lamellae depends on the illuminating wavelength; polarized light produces patterns aligned with the axis of polarization, and complex three-dimensional structures can be grown by changing the optical inputs during the growth. The spontaneous structuring of Se-Te films does not require the light source to be a laser, and occurs even when using light that is neither structured physically nor necessarily coherent.
We found that simultaneous illumination from two narrowband sources with different wavelengths results in a structure with a periodicity intermediate between that produced using either of the sources alone.3 The resulting periodicity could be varied by manipulating the relative intensity of the two sources. Simulations of agreed with experiments, and indicated that self-optimization of the pattern by the growing material resulted in maximization of the anisotropy of interfacial light absorption in the three-dimensional structure. We also found that simultaneous illumination from two linearly polarized sources with different directions of polarization results in a structure where the long axes of the lamellae are parallel to the intensity-weighted-average of the two polarization orientations.4 Illumination using two orthogonally polarized sources produced structures that consisted of two intersecting sets of orthogonally oriented lamellae. Simulations were consistent with experimental observations and showed that the lamellae preferentially absorb light polarized with electric field vectors parallel to the long lamellar axes.
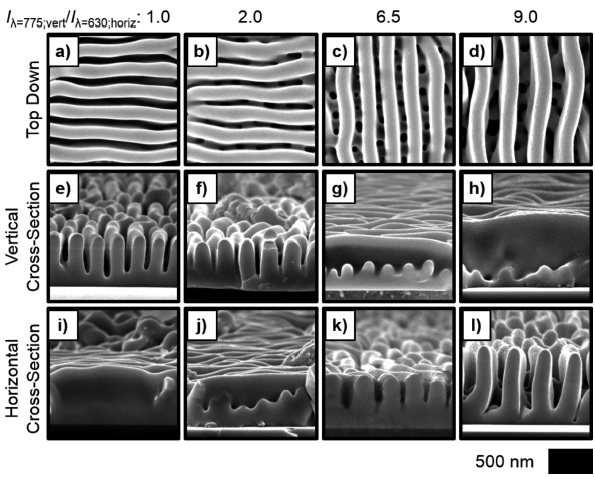
Scanning electron micrographs of selenium-tellurium films grown under simultaneous illumination from a horizontally polarized light source (λavg= 620 nm) and a vertically polarized light source (λavg=775 nm), as a function of relative intensity of the two sources. The relative heights of the two sets of intersecting lamellae depended on the relative intensities of the two light sources.4
References
- Sadtler, B.; Burgos, S. P.; Batara, N. A.; Beardslee, J. A.; Atwater, H. A.; Lewis, N. S., Phototropic growth control of nanoscale pattern formation in photoelectrodeposited Se-Te films. Proc. Natl. Acad. Sci. U.S.A. 2013, 110, 19707-19712.
- Carim, A. I.; Batara, N. A.; Premkumar, A.; Atwater, H. A.; Lewis, N. S., Self-Optimizing Photoelectrochemical Growth of Nanopatterned Se–Te Films in Response to the Spectral Distribution of Incident Illumination. Nano Lett. 2015, 15, 7071-7076.
- Carim, A. I.; Batara, N. A.; Premkumar, A.; Atwater, H. A.; Lewis, N. S., Polarization Control of Morphological Pattern Orientation During Light-Mediated Synthesis of Nanostructured Se-Te Films. ACS Nano 2015, 10, 102-111.