More energy from the Sun hits the Earth in one day than humans use in a decade.
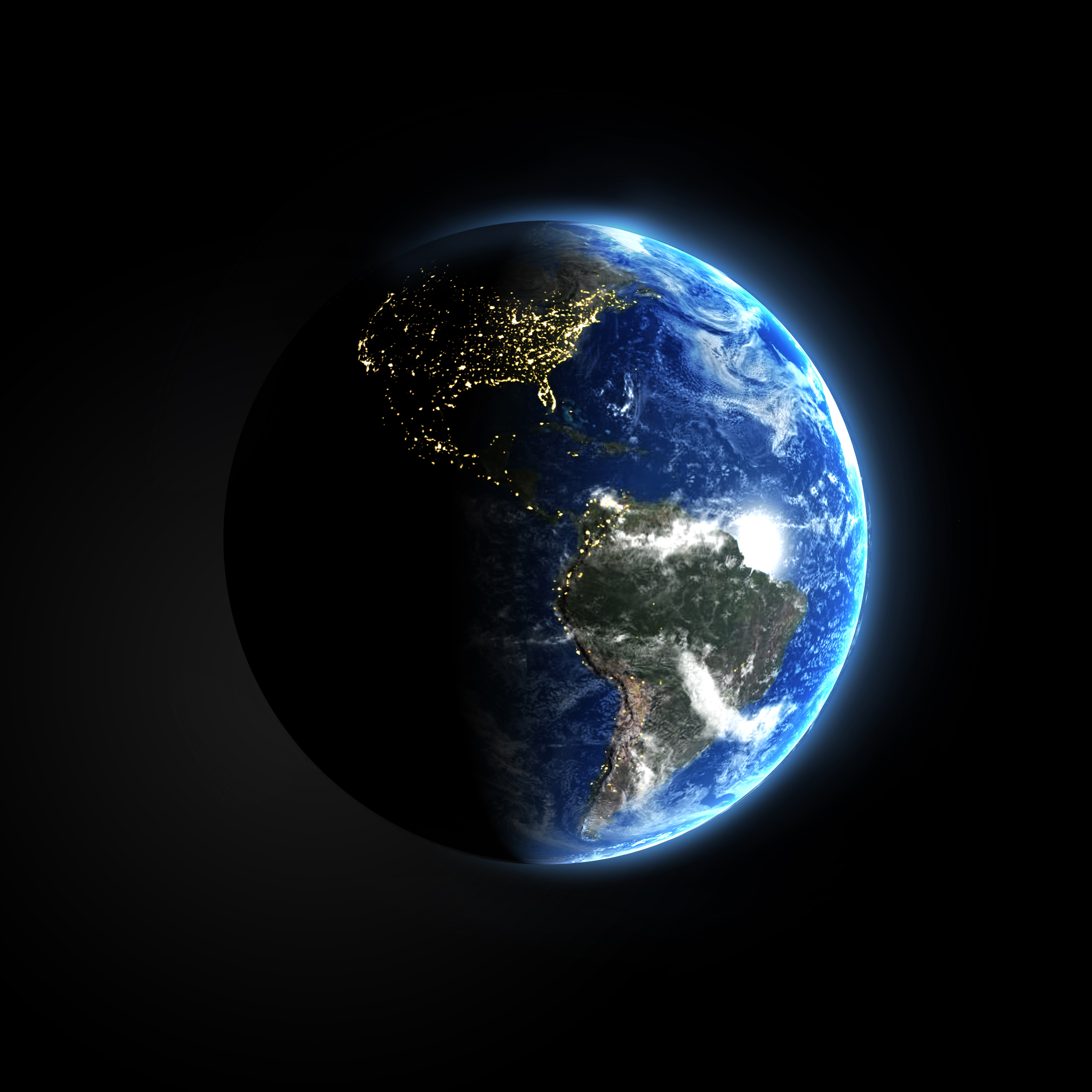
Making fuels from sunlight would store solar energy so it can be used on demand at any time of the day (or night).
Why Make Fuels from Sunlight?
Solar is by far the most abundant source of renewable energy available on Earth.
However, the sun is an intermittent and variable resource at any given location on the Earth’s surface. Therefore, a reliable energy system based on solar will require a way to store the energy captured from sunlight so that it will be available upon demand, at any time of day or year.
The chemical bonds found in fuels are the most dense way to store energy outside of an atomic nucleus. For example, the energy density of gasoline is between 65 and 100 times that of Li-ion batteries (0.46-0.72 MJ/kg: 65-100 tons of batteries would be needed to store the energy of 1 ton of gasoline.1
Fuels made from sunlight would:
- Provide the massive grid-scale energy storage that is needed to compensate for the intermittency of solar power;
Airplanes, ships, and heavy-duty trucks require energy-dense fuels.
- Provide an abundant source of the liquid fuels that are needed to power heavy-duty trucks, ships, and aircraft. Together these vehicles currently use ~40% of transportation fuels globally, and demand will grow further as global commerce expands especially in developing nations.
What is Solar Fuels Technology?
Solar fuels technologies use sunlight, water, carbon dioxide, and nitrogen from the air to produce fuels that can readily be used in our existing energy infrastructure. Solar fuels are sustainable and produce no net emissions of carbon dioxide.
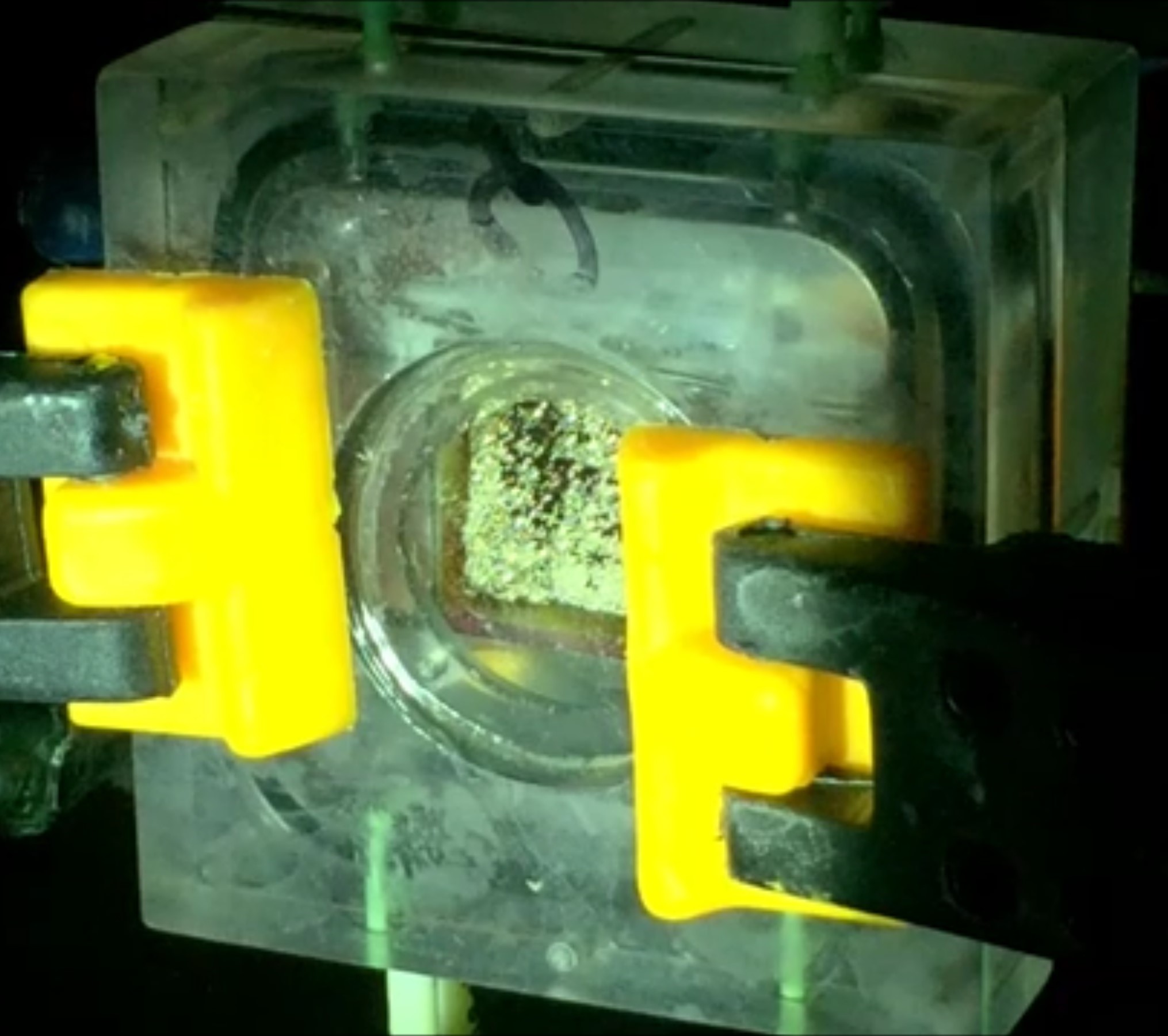
An example of solar fuels technology. Oxygen gas bubbles form at the center of the front of this water-containing cell when it is illuminated, and bubbles of hydrogen fuel form and are collected at the back of the cell. 3
Solar fuels technologies are analogous to natural photosynthesis – plants make fuels (biomass) from sunlight. The conversion of solar energy to biomass by plants can be up to 4.3% (C4 plants during the growing season).2 To be compatible with current infrastructure, the primary biomass made by plants – lignocelluose – must be converted into ethanol, biodiesel, or gasoline. This conversion requires energy and labor. Converting crops to fuels raises significant land-use concerns, specifically with regard to trading food for fuel.
What Fuels Can Be Made From Sunlight?
The Lewis Group has led the development of solar fuels technologies that produce hydrogen gas directly from sunlight and water. Carbon-containing fuels such as natural gas (methane) or liquid fuels such as methanol or ethanol might be produced from sunlight, water, and carbon dioxide. Ammonia for use as fertilizer in agriculture can be made indirectly from solar hydrogen or directly as a solar fuel from sunlight, water, and nitrogen in the air. Fuels can be readily interconverted using well-known processes.
Solar fuels would provide the same quality and quantity of energy services that end-users are used to, without a massive change in infrastructure, and hence would produce “drop-in” fuels that could serve critical sectors of the energy economy both in the developed and developing world.
Are Solar Fuels Technologies Ready for Commercialization?
Solar fuels technologies are developmental systems that are not yet ready for commercialization. A number of prototypes have been demonstrated but cannot compete with existing energy technologies nor provide long-term (20 years) stability.
What are the Key Hurdles?
The key hurdles to making fuels from sunlight commercially are:
- Cost competitiveness – Using current technologies, hydrogen made from sunlight would cost about ten times more than hydrogen made from fossil fuels.
- Materials discovery and system design – Fuels made from sunlight using current technologies cost so much more than fossil fuels, so radically new materials and system designs, that can be installed simply and at low costs are needed.
What are Scientists Doing to Overcome the Hurdles?
Several approaches are being pursued to construct a demonstration solar fuels system. In one approach, the molecular components of natural photosynthesis, such as chlorophylls, are synthesized and modified chemically to attempt to construct a complete, functional photosynthetic system in the absence of a living organism such as a plant or photosynthetic bacteria or algae. In another approach, inorganic molecules, such as transition metal complexes, are used instead of chlorophylls as the light absorbers, and these complexes are either coupled chemically to biological catalysts or are coupled to inorganic catalysts, such as metallic colloids or particles to generate solar fuels. In yet another approach, metal dyes are bonded to titania films to absorb light, and the dyes are also chemically coupled to transition metal catalysts to produce solar fuels. Inorganic semiconductors, similar to the ones used in solar panels, can also be used either indirectly to produce electricity in conjunction with catalysts for solar fuels production, or can be used as photoelectrodes to directly produce fuels from sunlight. All of the approaches have their own advantages and challenges both from a technical and cost perspective, requiring further materials discovery and research to address.
The Lewis Group is pursuing a single, one-step process that would produce fuels directly from sunlight, resulting in simple systems with low installation and materials costs. However, the integrated devices that can accomplish this single-step transformation of energy requires intimate contact between the materials that convert light to electricity (semiconductors) with the strongly corrosive environment needed for effective electrolysis. This causes semiconductors to be chemically attacked and to stop working. To address this problem, the Lewis Group is developing new synthetic strategies and materials to prevent semiconductor failure.
Common forms of commercial electrolyzers depend on expensive and scarce elements – platinum and iridium – to catalyze chemical reactions. The Lewis Group is working on integrating newly discovered inexpensive materials – made from elements plentiful on Earth such as nickel, cobalt, and phosphorous – as replacement catalysts to decorate photoelectrodes to enable direct solar-to-fuel transformations.
Where Can I Get More Information About Making Fuels from Sunlight?
- Check out the Lewis Group Solar Fuels Research page.
- Check out the Liquid Sunlight news feature by Katherine Bourzac in the Proceedings of the National Academy of Sciences of the United States of America.
References
- Haoyu Fang 2021 IOP Conf. Ser.: Earth Environ. Sci. 781 042023. DOI:10.1088/1755-1315/781/4/042023
- Robert E. Blankenship et al. Comparing Photosynthetic and Photovoltaic Efficiencies and Recognizing the Potential for Improvement. Science 332, 805-809 (2011). DOI:10.1126/science.1200165
- Verlage, E.; Hu, S.; Liu, R.; Jones, R. J. R.; Sun, K.; Xiang, C.; Lewis, N.; Atwater, H. A., A monolithically integrated, intrinsically safe, 10% efficient, solar-driven water-splitting system based on active, stable earth-abundant electrocatalysts in conjunction with tandem III-V light absorbers protected by amorphous TiO2 films. Energy Environ. Sci. 2015, 8, 3166-3172. DOI: 10.1039/C5EE01786F