Overview | Energy Systems | Solar Fuel Generation | Light Directed Growth
Goal
Aid in the planning of decarbonized electricity systems that are cost-effective, renewable, and reliable over decadal time scales.
Strategy
We use modeling and simulation tools to assess constraints and requirements – both geophysical and technoeconomic – which govern the technical and commercial feasibility of electricity systems dominated by renewable generation technologies.
We collaborate with Dr. Ken Caldeira from the Carnegie Institution for Science and Prof. Steven Davis from the University of California, Irvine, along with their respective group members, to identify projects, process geospatial data, run models, and analyze critical energy systems questions. Our collaboration unites numerous researchers with complementary skills, allowing us to produce holistic analyses related to Earth systems, energy, and climate.
Highlights
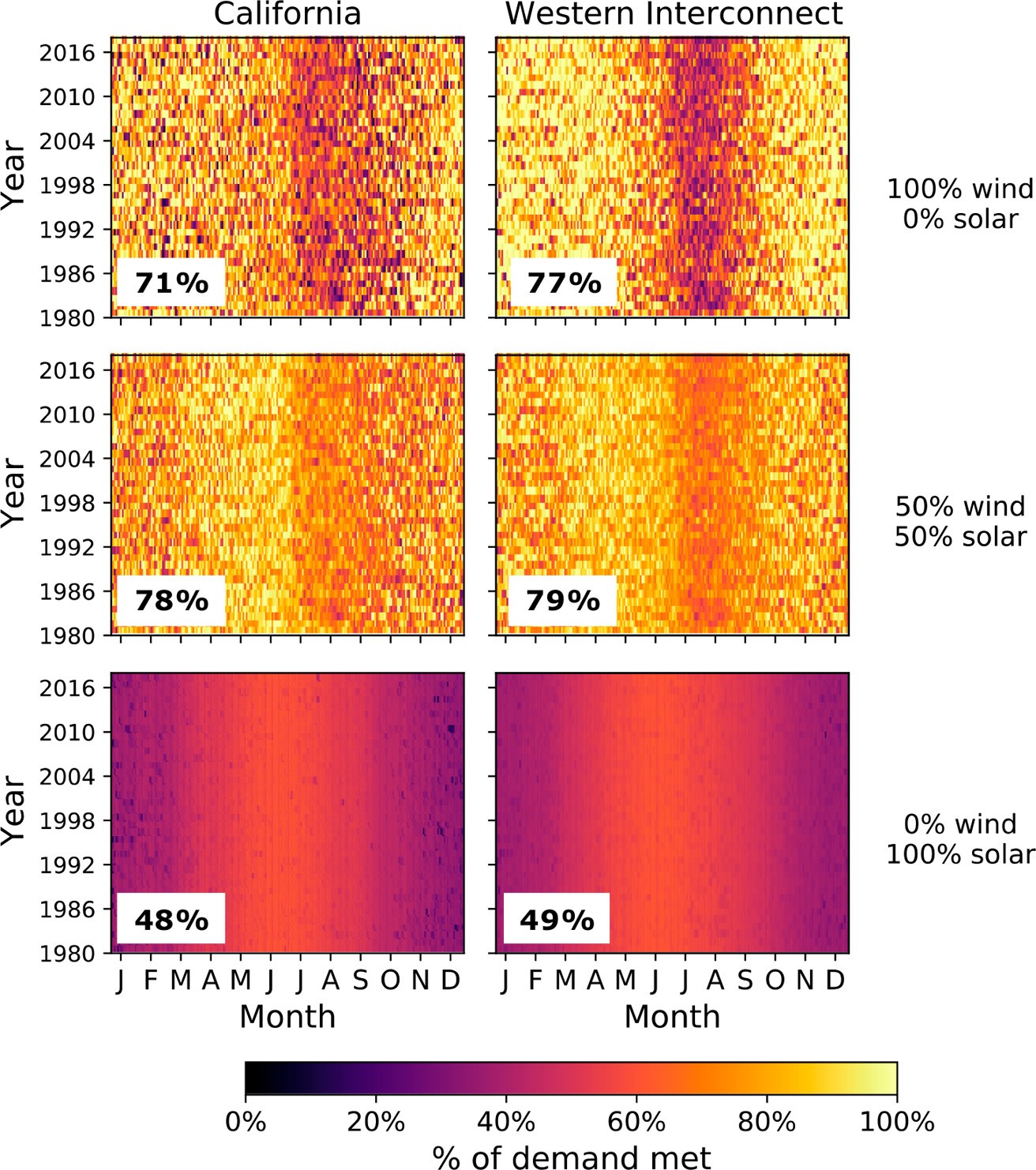
Percent demand met for each day over the 39-year period from 1980 to 2018 for wind and solar based electricity systems.
Wind and Solar Resource Droughts in California Highlight the Benefits of Long-Term Storage and Integration with the Western Interconnect1
This work highlights the necessity of considering the natural variability in wind and solar power as the U.S. fleet transitions to such variable renewable energy sources for electricity generation. The study focuses on California and the Western Interconnect (WECC), using hourly weather data across multiple decades along with historical electricity demand data to examine the discrepancies between wind and solar power supply and electricity demand.
In this article, we introduce the concept of “resource droughts”, defined as days when the daily power from wind or solar was less than half of the 39-year daily mean for that day of the year. Over a 39-year average, California experienced 6.6 days of solar drought and 48 days of wind drought annually, whereas the WECC experienced significantly fewer droughts, with 0.41 days for solar and 19 days for wind.
We evaluate the effectiveness of long-term storage solutions and the benefits of geographically diversifying generation resources to reduce system costs. For example, meeting California’s electricity demand using generation resources from the entire WECC could reduce costs by 9% compared to relying solely on resources within California.
Finally, incorporating long-duration storage could further lower system costs by 21% for consideration of California as an isolated system.
Role of Long-Duration Energy Storage in Variable Renewable Electricity Systems2
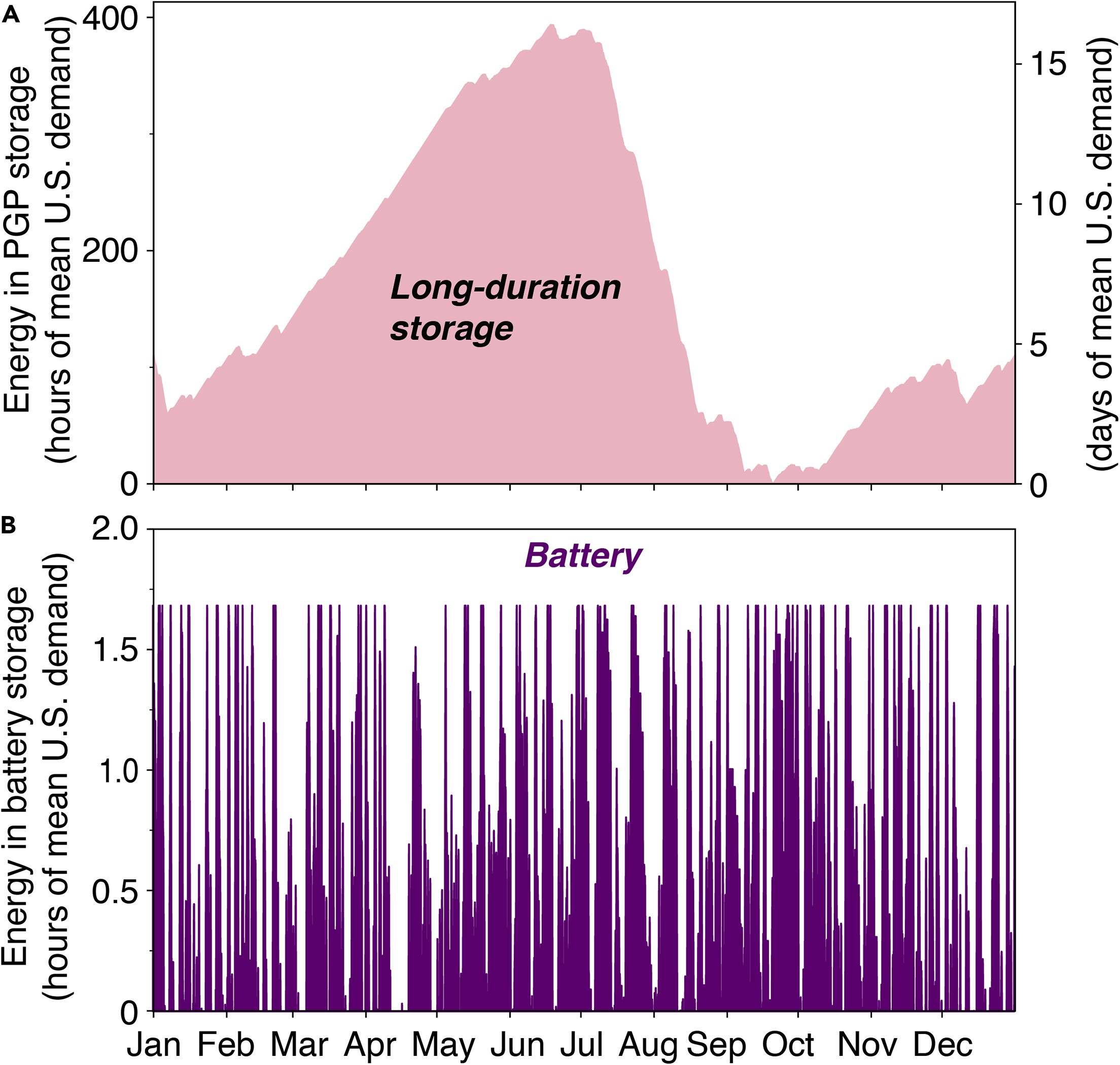
Usage of long term and short term storage technologies
This article investigates an electricity systems model over a multi-year timescale to reveal that long-duration storage (>10 h) can fill unique roles compared to battery storage, like seasonal and even multi-year storage, making it valuable to least-cost electricity systems.
Importantly, we found that long-duration storage reduces costs of wind-solar-battery systems. The utilization and benefits of long-duration storage increase when the system is planned with more years of data (and therefore the system must deal with more weather variability and extreme events).
We show that variable renewable power systems are much more sensitive to reductions in long-duration storage costs than to equal reductions in battery costs. Indeed, long-duration storage cost reductions lower system costs 2× more than batteries.
These results suggest that large-scale deployment of long duration storage (e.g. hydrogen power-to-gas-to-power, compressed air, and pumped hydro) and cost improvements in such technologies may greatly reduce the cost of future variable renewable electricity systems.
References
- Rinaldi, K. Z. et al. Wind and Solar Resource Droughts in California Highlight the Benefits of Long-Term Storage and Integration with the Western Interconnect. Environmental Science and Technology, 2021, 55, 9, 6214-6226.
- Dowling, J. A. et al. Role of Long-Duration Energy Storage in Variable Renewable Electricity Systems. Joule, 2020, 4, 1907-1928.