How Does The Lewis Group Approach Making Fuels from Sunlight?
In order to radically reduce the costs of making fuels from sunlight, we focus on developing single-step devices from Earth-abundant elements, using techniques which minimize the total costs of materials without compromising on efficiency or safety. Since capital costs – for example labor, wiring, and equipment – need to be reduced significantly for solar fuels to be cost-competitive with fossil fuels, we focus on an architecture compatible with flexible materials, and envision a system that can be installed simply, like unrolling bubble wrap or artificial turf.
What Might a Solar Fuels Device Look Like?
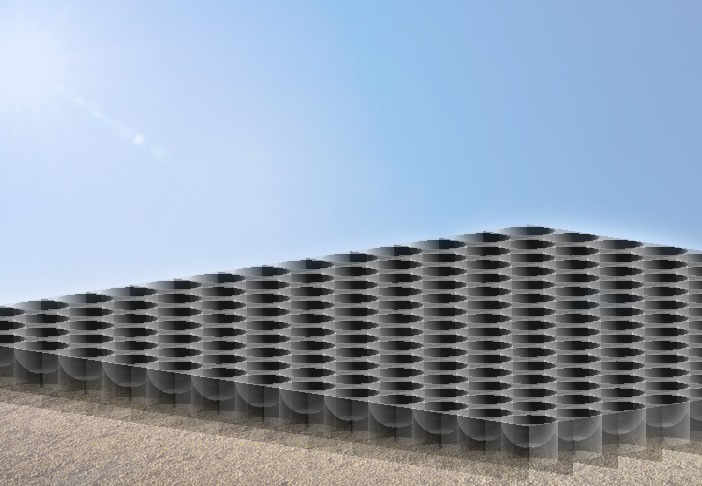
Bubble-wrap design for a solar fuels device, consisting of plastic cells that concentrate sunlight, with each cell containing a small active component (semiconductors, catalysts, and membranes) and water.
We are designing systems that can make fuels from sunlight efficiently and safely. We use modeling and simulation tools to evaluate device designs so that we understand the theoretical limits to the design, and so that we understand which parameters have the greatest impact on performance. Much of this modeling is done in collaboration with the team of Chengxiang Xiang, a Lewis Group alumnus and a Principal Investigator with the Joint Center for Artificial Photosynthesis.
The bubble-wrap design sketched on this page consists of an array of plastic cells, each of which contains a small active component and water. This design uses simple, inexpensive plastic lenses to concentrate sunlight, and therefore allows a ten-fold reduction in the materials used for the active components relative to the materials requirements for devices without concentrating lenses. Since most of the design is plastic, the materials costs would be low, and the structure would be flexible, allowing it to be rolled out like bubble wrap or artificial turf, radically reducing installation costs relative to current solar fuels technologies. Other designs, for example an array of long cells based on trough-shaped plastic lenses, would achieve comparable efficiency while reducing materials and installation costs.
The optimal designs (on cost and efficiency metrics) vary depending on whether the device makes hydrogen from sunlight and water or carbon-based fuels from carbon dioxide, water, and sunlight. Devices designed to produce hydrogen fuel typically contain either acidic or alkaline water, while those designed to produce carbon-based fuels typically contain water buffered with carbonate salts. Some designs allow cells to fed either by humidified air, or by humidified deoxygenated air.
The active components of a solar fuels device are light absorbers and catalysts. For safety and efficiency purposes, solar fuels devices also need separators to prevent mixing of the chemical products of the reaction (fuels and oxygen).
What Might the Active Components Look Like?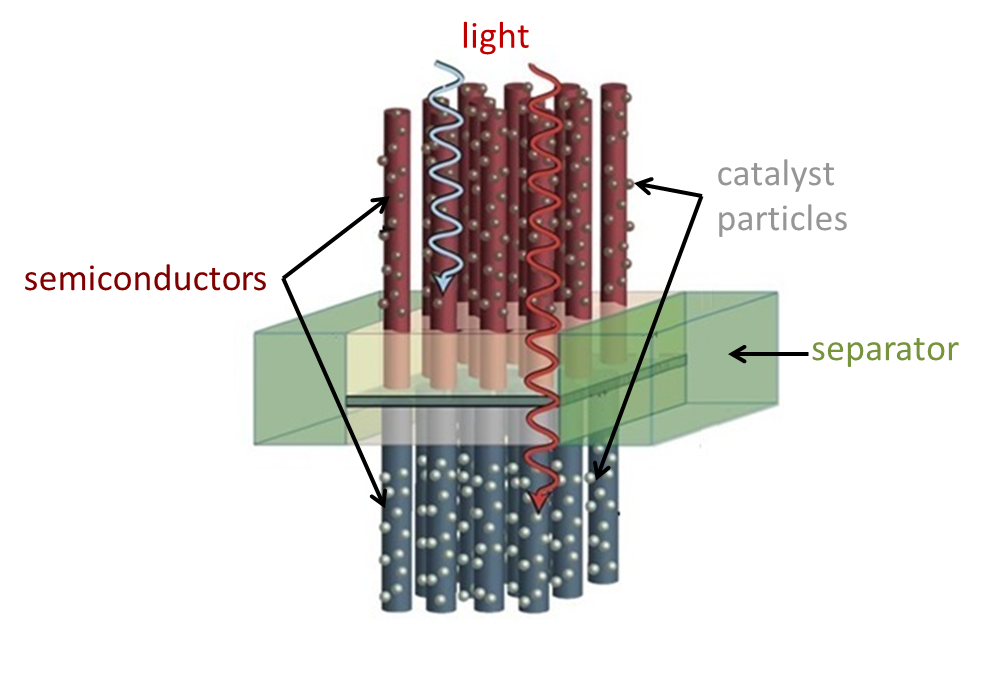
We envision active components consisting of wire-shaped semiconductors that are decorated with catalyst particles and embedded within a separator.
Semiconductors
The function of the semiconductors is to absorb sunlight and convert the energy in the absorbed sunlight into the potential energy of separated positive and negative charges.
Since sunlight is multispectral – it contains a wide range of energies (higher energies for violet and blue, lower energies for red) – we envision two complementary semiconductors, one on top which absorbs only higher energy light, and the other on the bottom to absorb any light which passes through the top (lower energies).
We can use silicon as the bottom absorber, but we are looking for a semiconductor for the top absorber that will not corrode and that can be made inexpensively from Earth-abundant elements.
Catalysts
The active component needs two catalysts: one for the chemical reaction that yields fuels, and the other for the chemical reaction that yields oxygen gas. The reaction that forms oxygen gas is a necessary partner to the fuel-forming reaction, providing the electronic charges needed for the fuel-forming reaction, and closing a combustion-based fuel cycle.
Without catalysts, these reactions would proceed very slowly (if at all). Although the reactions are thermodynamically favored under the operating conditions in a solar fuels device, the reactions first need an uphill push – for example, electronic charges and molecules need to get close enough to react. Catalysts increase the reaction rates by decreasing the height of the hill.
Catalysts used in devices which make fuels from sunlight need long-term stability, preferably decades of stability under operating conditions. The catalysts need to attach firmly to the semiconductors, and can’t dissolve, corrode, or undergo any other chemical reactions that would reduce their effectiveness. Stable catalysts are often based on expensive and rare elements – platinum, iridium, ruthenium and rhodium are a few examples. We are looking for catalysts based on Earth-abundant elements, such as nickel, iron, cobalt, or zinc. Ideally the catalysts would be transparent to sunlight, so that they don’t block light from reaching the semiconductors; however, we have developed strategies for catalyst placement and loading that would considerably reduce the amount of light blocked by an opaque catalyst.
Several catalysts based on Earth-abundant elements and capable of making hydrogen fuel from water have been discovered as a result of solar fuels research. This set of catalysts – transition metal phosphides and nickel-molybdenum – offers options for stability and efficiency for either acidic or alkaline water. We also have catalysts – nickel-iron oxide and cobalt oxide – that offer stability and efficiency for making oxygen from alkaline water.
We are looking for catalysts that can make carbon-based fuels from carbon dioxide and water. We are also looking for catalysts that can make oxygen from acidic water. We collaborate with Professor Ray Schaak at the Pennsylvania State University for many of our catalyst-discovery efforts.
Separators
Separators are critical components in a solar fuels device. For safety reasons, separators must prevent the fuels and oxygen formed by solar fuels devices from mixing in the cell or in the output streams. In order for the devices to function, separators must also allow ionic current to pass.
Without a separator ionic current would pass, but the products (fuels and oxygen) would not readily be separated. If fuels and oxygen are not separated everywhere and all the time: 1) The products from desired chemical reactions – fuels and oxygen – will recombine yielding the starting materials while releasing, possibly explosively, the energy of sunlight that was stored as chemical bonds; and, 2) Reverse chemical reactions will occur that convert fuels back to water (and carbon dioxide for carbon-based fuels) and that convert oxygen back to water, wasting the energy from sunlight that had been stored in the chemical bonds of the fuel.
Where Can I Get More Information on Solar Fuels Research?
- Check out Who is Making Fuels from Sunlight?
- Check out the Lewis Group Research pages.
- Read “Research Opportunities to Advance Solar Energy Utilization” published in Science (volume 351, 2016) and written by Prof. Nate Lewis.
- Read “Will Solar-Driven Water-Splitting Devices See the Light of Day” published in Chemistry of Materials (volume 26, 2014, pages 407–414) and written by Lewis Group alumnus James McKone, Prof. Harry Gray, and Prof. Nate Lewis.
by Kimberly Papadantonakis, June 2016.